Ethics code: Not applicable
Sheir M, Mohammed N, Farroh K. Production and Evaluation of Chitosan-Collagen Nanocomposite Packaging Film from Chicken Feet Waste. J. Food Qual. Hazards Control 2025; 12 (1) :61-72
URL:
http://jfqhc.ssu.ac.ir/article-1-1242-en.html
Department of Special Food and Nutrition, Food Technology Research Institute, Agricultural Research Center, Giza, Egypt , Marwa.sheir@yahoo.com
Full-Text [PDF 644 kb]
(170 Downloads)
|
Abstract (HTML) (167 Views)
- Chicken feet, a common poultry by-product, are a rich source of collagen.
- Acetic acid (5%) was identified as the ideal solvent and highly effective for collagen extraction.
- Hydrolyzing chicken feet collagen has proven to be a successful method for producing chitosan-collagen nanocomposite film.
- Chitosan-collagen nanocomposite film is ideal for packaging animal-based foods or high-fat products.
Full-Text: (33 Views)
Production and Evaluation of Chitosan-Collagen Nanocomposite Packaging Film from Chicken Feet Waste
M.A. Sheir ** , N.S. Mohammed 2, K.Y. Farroh 3
1. Department of Special Food and Nutrition, Food Technology Research Institute, Agricultural Research Center, Giza, Egypt
2. Department of Meat and Fish Technology Research, Food Technology Research Institute, Agricultural Research Center, Giza, Egypt
3. Nanotechnology and Advanced Materials Central Lab., Regional Center for Food and Feed, Agricultural Research Center, Giza, Egypt
HIGHLIGHTS
- Chicken feet, a common poultry by-product, are a rich source of collagen.
- Acetic acid (5%) was identified as the ideal solvent and highly effective for collagen extraction.
- Hydrolyzing chicken feet collagen has proven to be a successful method for producing chitosan-collagen nanocomposite film.
- Chitosan-collagen nanocomposite film is ideal for packaging animal-based foods or high-fat products.
Article type
Original article |
|
ABSTRACT
Background: Annually, approximately 3.9 million metric tons of chicken feet are produced. As a common poultry by-product, they are a rich collagen source, representing over 40% of total amino acids. To maximize their potential, innovative utilization is required to enhance their value. The study aimed to investigate the use of broiler chicken feet for producing acid-hydrolyzed collagen and incorporating it into chitosan-collagen composite films, including nanocomposite films.
Methods: Chitosan film, chitosan-collagen composite film, and chitosan-collagen nanocomposite film were prepared. Physical, mechanical, antioxidant, and antimicrobial properties of these composite films were evaluated and compared to identify the most suitable material for food packaging.
Results: Chitosan-collagen nanocomposite film demonstrated favorable characterizations, including mechanical, physical, antioxidant, and antimicrobial properties, in addition to lower oxygen permeability than other films.
Conclusion: Chitosan-collagen nanocomposite film is suitable as a packaging material for preservation purposes, especially in animal-based or high-fat foods.
© 2025, Shahid Sadoughi University of Medical Sciences. This is an open access article under the Creative Commons Attribution 4.0 International License. |
Keywords
Chitosan
Collagen
Food Packaging
Nanocomposites. |
|
Article history
Received: 07 Jul 2024 Revised: 31 Oct 2024 Accept: 02 Feb 2025 |
|
Abbreviations
DLS=Dynamic Light Scattering
DPPH=2,2-Diphenyl-1-Picrylhydrazyl
HR-TEM=High Resolution Transmission Electron Microscope
IC50=Half-Maximal Inhibitory Concentration Measurements
OTR=Oxygen Transmission Rate
XRD=X-Ray Diffraction |
|
To cite: Sheir M.A., Mohammed N.S., Farroh K.Y. (2025). Production and evaluation of chitosan-collagen nanocomposite packaging film from chicken feet waste. Journal of Food Quality and Hazards Control. 12: 61-72.
Introduction
Chitosan is a linear copolymer polysaccharide of β-linked D-glucosamine and N-acetyl-D-glucosamine. It is mainly produced by deacetylation of chitin, which is mainly derived from shrimp shells and other crustaceans. Due to its biodegradability, biocompatibility, excellent film formation, availability, antimicrobial properties, and cationic nature, chitosan is an excellent choice as a polymeric matrix for novel applications like food packaging (Haghighi et al., 2020; Wang et al., 2018). However, its weak mechanical and barrier properties pose a limitation. These properties can be enhanced by forming hydrogen bonds between the hydroxyl groups of chitosan and natural polymers via amine groups (Momtaz et al., 2024).
Collagen stands as the predominant protein in vertebrates, making up around 25% of all the proteins in them (Amirrah et al., 2022). This particular protein is distinguished by its fibrous structure, ability to naturally decompose, and film formation capability (Hashim et al., 2014). Collagen is mostly found in the extracellular matrix of connective tissue, such as cartilage, bones, tendons, ligaments, and skin. It consists of three alpha-polypeptide chains organized in a triple helix configuration. Reticulate fibrils are formed through the association of these chainsand further combine to create solid fibers. Due to their triple solid helix structure, these fibers are essential for the extracellular matrix's endurance and high mechanical strength. Moreover, collagen is a good contender for gel formation, texturizing and thickening due to its high capacity for water absorption. It is widely utilized in industries such as leather, and film materials, cosmetics, biomedicine, pharmaceuticals, and food production (Amirrah et al., 2022; Hashim et al., 2014).
The poultry processing industry produces 3.9 million metric tons of chicken feet each year. Chicken feet are a rich source of collagen and despite being one of the main poultry by-products, they are exported as food in some regions of the world (Fatima et al., 2022; Ozturk-Kerimoglu., 2023.). Chicken feet are mainly composed of bones, tendons skin, and cartilage. Broiler meat contains more than 40% of the total amino acids present including essential amino acids such as lysine, arginine, leucine, and histidine (Dalle Zotte et al., 2020; Santana et al., 2020). Chicken feet contain a high percentage of proteins, primarily collagen, which can be utilized in various applications. However, the presence of fat and other concomitant substances poses challenges (Mokrejs et al., 2017). This study focuses on producing chitosan-collagen nanocomposite food packaging films using broiler chicken feet waste as a collagen source, aligning with Egypt's zero-waste strategy. This research contributes to sustainability goals by promoting economic well-being, social welfare, and environmental protection.
Materials and methods
Sample collection
At the end of October 2023, 10 kg fresh chicken feet were purchased from the local market, Giza, Egypt, and were transported to a laboratory in an ice box. The samples were de-nailed manually, washed with tap water, and kept at 20 ºC. Chitosan (viscosity: 20-300 cP, molecular weight: 50,000-190,000 Da, and degree of deacetylation 75-85%). All chemicals and solvents used in this investigation were obtained from Sigma-Aldrich, USA.
Preparation of collagen from chicken feet using 5% acetic acid hydrolysis
The extraction was carried out using the method obtained from Liu et al. (2001) with slight modifications. After the chicken feet were deboned and chopped into small (5 cm) pieces, a mincer (Philips, Cucina Series, Shanghai, China) was used to grind them. The minced chicken feet were demineralized using 5% w/v acetic acid (chicken feet/solution: 1/8) for 48 h at 4-7 °C. After soaking, a blender (31BL91 ,Warning Blender, USA) operating at 10,000 rpm (45 s work and 15 s rest) was used for 5 min to homogenize the suspended chicken feet solution. To remove bone residues, the sample was filtered using a stainless steel filter. The filtrate was neutralized to pH 7 with 0.1 m NaOH and centrifuged at 8,000 g for 15 min at 10 °C. Half of the precipitates were collected to obtain a collagen solution for the preparation of chitosan-collagen composite and Chitosan-collagen nanocomposite film. The remaining half was lyophilized using a freeze dryer (LD53, Kingston, New York), and the resulting collagen powder was weighed and recorded. The pH of the crude collagen sample was measured following the procedure of Okerman (1984). The yield of isolated collagen was calculated on a dry weight basis according to Chuaychan et al. (2015), while the swelling percentage of dry collagen was determined using the method described by Ananth et al. (2024).
Preparation of chitosan film and chitosan-collagen composite
Chitosan film and chitosan-collagen composite were prepared using wet chemistry methods. Chitosan film was prepared by dissolving one g of chitosan powder in acetic acid (1% v/v). To prepare chitosan-collagen composite, the chitosan aqueous solution (1% w/v) was prepared by dissolving chitosan in acetic acid solution (1% v/v) at room temperature. Subsequently, the collagen solution (1% w/v) was prepared by dissolving collagen in acetic acid solution (1% v/v) at room temperature. The collagen solution was then added to the chitosan nanoparticle solution in the presence of Tween 80 with vigorous stirring. To prepare the film sheet, both solutions were cast on a 24 cm by 30 cm leveled glass plate separately, covered with Teflon film, and allowed to dry for 48 h at room temperature (23±2 °C). Once dried, the films were peeled off from the glass plate.
Preparation and characterization of chitosan-collagen nanocomposite film
Chitosan-collagen nanocomposite film was prepared following Sharkawy et al. (2021) with minor modifications. A 1% (w/v) aqueous solution of chitosan was prepared by dissolving chitosan in a 1% (v/v) acetic acid solution at ambient temperature. Sodium tripolyphosphate (STPP) was dissolved in 10 ml of deionized water to a final concentration of 0.3 mg/ml as a cross-linking agent. Collagen was dissolved in a 1% (v/v) acetic acid solution at room temperature to create a 1% (w/v) collagen solution. The collagen solution was then added to the chitosan nanoparticle solution in the presence of Tween 80 as a surfactant to reduce the hydrodynamic diameter of the nanoparticles, followed by a 5 min ice bath at 50% amplitude to prevent overheating (UP400ST, Hielscher, Germany). The film solutions were cast onto a leveled glass plate (24×30 cm), covered with Teflon film, and dried for 48 h at room temperature (23±2 °C). The dried films were peeled off from the glass plate. The chemical structure of chitosan-collagen nanocomposite film was assessed using X-Ray Diffraction (XRD) technique. The corresponding XRD pattern was recorded in the scanning mode (X ’pert PRO, PAN analytical, Netherlands) operated by Cu K radiation tube (=1.54 A˚) at 40 kV and 30 mA. The obtained diffraction pattern was interpreted by the standard International Center for Diffraction Data (ICDD) library installed in PDF4/2023 software. Dynamic Light Scattering (DLS) measurement of size and zeta potential was undertaken using a nano-zeta sizer (Malvern, ZS Nano, UK). Morphological analysis of chitosan-collagen nanocomposite film was conducted via High Resolution Transmission Electron Microscope (HR-TEM; Tecnai G2, FEI, Netherlands) operating at an accelerating voltage of 200 kv. To reduce the particles aggregation, the diluted chitosan-collagen nanocomposite film solution was ultra-sonicated for 5 min. Using micropipette, three drops of the ultra-sonicated solution were pipetted on a carbon coated-copper grid and left to dry at room temperature. HR-TEM imaging provided detailed insights into the morphology of the deposited nanocomposites. All synthesis and characterization of chitosan-collagen nanocomposite film were carried out at the Nanotechnology and Advanced Materials Central Laboratory (NAMCL), Agricultural Research Center (ARC), Giza, Egypt.
Physical and mechanical analysis
-Color analysis and transparency (%)
A colorimeter was used to measure the average color value of each film according to Azizah et al. (2023), with data represented by the values L* (lightness-darkness), a* (red-green), and b* (yellow-blue). A spectrophotometer was used to test the transparency of edible films at a wavelength of 600 nm (λ). Samples were cut into 4×1cm sizes, and placed in cuvettes. A spectophotometer (UV-1201v, Shimadzu, Japan) was used to obtain transmission data (T600), which were then recorded and calculated according to Ningrum et al. (2021).
-Thickness, tensile strength, and elongation
A digital micrometer was used to measure the thickness of each film, following the method described by Ningrum et al. (2021), The tensile strength and elongation of the films were estimated according to Ningrum et al. (2021). The reported results represent the average of four measurements taken for each film.
-Water Vapor Transmission Rate (WVTR) and Oxygen Transmission Rate (OTR)
The OTR and WVTR for the samples were measured using the procedures outlined by Youssef et al. (2021). Assessments were performed in triplicate for each film, with the average results documented.
-Moisture content, water solubility, and Swelling index
The moisture content was determined according to Hromiš et al. (2016), while the solubility of each edible film was measured using the dry matter of the film solubilized in water for 24 h, following Ningrum et al. (2021). The swelling index was assessed and calculated according to Delavari et al. (2022).
In Vitro Antioxidant activity using 2,2-Diphenyl-1-Picrylhydrazyl (DPPH) radical scavenging assay
Twenty-five mg of each film were homogenized with ethanol for 5 min at 10,000 rpm and then centrifuged for 10 min at 3,000 rpm. A DPPH solution was prepared by dissolving 0.1 mm DPPH in 2.0 ml of ethanol. One ml of this solution was added to three ml of different film extracts in ethanol at various concentrations (3.9, 7.8, 15.62, 31.25, 62.5, 125, 250, 500, 1,000 μg/ml). Only films soluble in ethanol were used, and different concentrations were prepared using a dilution method. The mixture was vigorously shaken and allowed to stand at room temperature for half an hour. Absorbance was measured at 517 nm using a UV-VIS Milton Roy spectrophotometer (Milton Roy, New York, USA). The experiment was conducted in triplicate with ascorbic acid as the reference standard. The half-maximal Inhibitory Concentration (IC50) value, representing the concentration required to inhibit 50% of the DPPH free radical, was calculated using a log dose inhibition curve. Lower absorbance indicated higher free radical scavenging activity (Castaldo et al., 2021; González-Palma et al., 2016).
In vitro antimicrobial activity testing
The agar diffusion method was employed to assess the antimicrobial properties of edible films against four bacterial strains (Bacillus subtilis, Staphylococcus aureus, Salmonella typhi, and Pseudomonas aeruginosa) and two fungi (Candida albicans and Mucor circinelloides). The films were cut into 10 mm diameter disks using a hole puncher (Zs Enterprise Co., ltd Dongguan, Guangdong, China) and placed on microbial cultures. Gentamycin served as a positive control for bacterial strains, while fluconazole was used for fungal strains. Fungal cultures were incubated at 25-30 °C for 3 to 7 days, and bacterial cultures were incubated at 37 °C for 24 h. Triplicate plates were prepared for each test. Antimicrobial activity was evaluated by measuring the zone of inhibition based on the method described by Abd-Alhadi et al. (2023), with slight modifications.
Statistical analysis
Statistical analysis was performed using SPSS, version 25 (IBM Corp., released in 2013) by One-Way ANOVA. Data were analyzed following a complete randomization design (Steel et al., 1997). Multiple comparisons were conducted using the Duncan test with a significance level set at p<0.05.
Results and discussion
The data presented in Table 1 summarize the results for pH, swelling, yield, and collagen%, which were found to be 3.63, 229.2, 28.87, and 16.99%, respectively.
The physicochemical characterization of the produced chitosan/collagen nanocomposite film is displayed in Figure 1, utilizing various analytical methods. Figure 1A shows the X-ray powder diffraction patterns of chitosan/collagen nanocomposite film, which exhibits no sharp peaks. The polymer chains form a dense network structure of chitosan nanoparticles due to cross-linking by STPP. The XRD pattern reveals a broad, typical hump starting from 2θ=13.6 ° to 2θ=39 °, indicating an amorphous structure. Figures 1B and 1C present the particle size distribution curve derived from DLS data. The DLS analysis showed a particle size of 43.8 nm for chitosan/collagen nanocomposite film, with the surface charge or zeta potential of +39.9 mv. Figure 1D, an HR-TEM image, illustrates the nanoparticles' roughly spherical shape, smooth surface, and size range of approximately 38.8 nm.
The color of food packaging materials is a crucial factor affecting consumer acceptance and the commercial success of food products. The appearance and color characteristics (L*, a*, and b*) are presented in Figure 2 and Table 2. Pure chitosan film exhibited high lightness values (60.9), but incorporating collagen reduced brightness, with the L* value decreasing to 52.9. However, the incorporation of chitosan with collagen nanoparticles in films, reduced L* value to 56.5, likely due to better distribution of chitosan/collagen nanoparticles in the film matrix. A significant increase in a* and b* parameters was observed in chitosan/collagen composite film, followed by chitosan/collagen nanocomposite film, compared to neat chitosan film. Appearance and transparency are critical properties for biopolymeric films, especially when used as food coatings, as they enhance visibility and thereby attractiveness. However, designing food packaging materials requires balancing light-blocking properties and translucency. Among single-polymer films, chitosan film demonstrated the best barrier properties against UV light, with a transmittance (T) of 1.503% (Table 2). For comparison, the transparency values of chitosan/collagen composite and chitosan/collagen nanocomposite films were 0.847 and 1.15%, respectively. For practical applications of edible films, they must not be heavily tinted or discolored to avoid affecting the final product's appearance on display. As shown in Figure 2, the obtained films had no cracks or visual defects.
A statistical comparison was conducted on the mechanical properties of the films (Table 3). According to the data, chitosan/collagen composite film had the highest thickness (0.083±0.003 mm), while chitosan/collagen nanocomposite film were the thinnest (0.053±0.003 mm) compared to chitosan film (0.063±0.003 mm). Table 3 also indicated a significant (p<0.05) variation in elongation percentage among film types, with chitosan/collagen nanocomposite film having the highest elongation (2.73%), followed by chitosan film (2.23%), and chitosan/collagen composite film (1.59%). Additionally, neat chitosan film showed high tensile strength values (35.47 MPa), whereas their composite or nanocomposite had significantly lower tensile strength values (ranging from 13.71 to 11.5 MPa).
As displayed in Table 4, chitosan/collagen nanocomposite film exhibited lower water vapour barrier properties and then chitosan/collagen composite film and chitosan film. Additionally, Table 4 indicated that the lowest oxygen transmission rate was recorded for chitosan/collagen nanocomposite film, followed by chitosan/collagen composite film, and then chitosan film.
As detailed in Table 5, chitosan film displayed the highest moisture content and water solubility, followed by chitosan/collagen composite film and then chitosan/collagen nanocomposite film. During the swelling index study, the results revealed that collagen and chitosan as edible films showed significant differences in the swelling index (p<0.05). A significant reduction in the swelling index was observed for chitosan/collagen nanocomposite film compared to chitosan/collagen composite film.
As observed in Figure 3 and Table 6, chitosan/collagen nanocomposite film exhibited the highest antioxidant activity among the samples, with a significant increase in DPPH percentage to 92.60% and an IC50 value of 16.76 μg/ml. This was followed by chitosan/collagen composite film and chitosan film, which showed DPPH percentages of 89.95 and 67.83%, and IC50 values of 19.57 and 205.95 μg/ml, respectively.
The antimicrobial activity values of the edible films, compared with gentamicin as a control for bacteria and fluconazole for fungi, are presented in Table 7 and Figure 4. The results indicated that chitosan film did not exhibit any inhibition zone.
Furthermore, our findings showed that chitosan/collagen nanocomposite film demonstrated superior antibacterial activity against B. subtilis (ATCC 6633), S. aureus (ATCC 6633), and P. aeruginosa (ATCC 90274) compared to gentamicin and effectively inhibited Candida albicans (ATCC 10221) growth compared to fluconazole followed by chitosan/collagen composite film. Additionally, chitosan/collagen composite film exhibited a larger inhibition zone against S. typhi (ATCC 6539), recording 25±0.06 mm, compared to gentamicin (20±0.56 mm) and chitosan/collagen nanocomposite film (18±0.18 mm). Our results also indicated that neither chitosan/collagen composite film nor chitosan/collagen nanocomposite film showed inhibition zones against M. circinelloides (AUMMC 11656).
Table 1: Physicochemical properties of acid hydrolyzed collagen (Values are Mean±SD)
Parameters |
Acid hydrolysis collagen (n=6) |
pH |
3.63±0.12 |
Swelling (%) |
229.2±1.39 |
Yield (%) |
28.87±1.60 |
Collagen (%) |
16.99±0.55 |
Figure 1: Chitosan-collagen nanocomposite film characterization
(A): X-Ray Diffraction (XRD) pattern analysis; (B): Particle size distribution of synthesized chitosan-collagen nanocomposite film, showing an average size of 43.8 nm; (C): Zeta potential measurement of the produced chitosan-collagen nanocomposite film, indicating a surface charge of +39.9 mV; (D): High-Resolution Transmission Electron Microscopy (HR-TEM) image illustrating the generated chitosan-collagen nanocomposite film with an average particle size of 38.8 nm and a nearly spherical shape
Figure 2: Images of chitosan film (CH), and chitosan-collagen composite film (CH/CO), and chitosan-collagen nanocomposite film (NCH/NCO)
Table 2: Color parameters (L*, a*, and b*) and transparency% of the tested film formulations
Treatment |
Color parameters |
Transparency% |
L* |
a* |
b* |
Chitosan film (n=6) |
60.960±0.012 a |
0.893±0.003 c |
2.423±0.033 c |
1.503±0.023 a |
Chitosan-collagen composite film (n=6) |
52.967±0.017 c |
1.663±0.033 a |
8.093±0.003 a |
0.847±0.022 c |
Chitosan-collagen nanocomposite film (n=6) |
56.567±0.217 b |
1.177±0.003 b |
5.903±0.033 b |
1.15±0.021 b |
Values are Mean±SD. There is no significant difference (p>0.05) between any two means within the same column that share the same superscript letter.
Table 3: Mechanical properties of films
Treatment |
Thickness (mm) |
Elongation (%) |
Tensile strength (MPa) |
Chitosan film (n=6) |
0.063±0.003 b |
2.233±0.033 b |
35.470±0.040 a |
Chitosan-collagen composite film (n=6) |
0.083±0.003 a |
1.587±0.037 c |
13.713±0.033 b |
Chitosan-collagen nanocomposite film (n=6) |
0.053±0.003 b |
2.733±0.033 a |
11.153±0.033 c |
Values are Mean±SD. There is no significant difference (p>0.05) between any two means within the same column that share the same superscript letter.
Table 4: Water Vapor Transmission Rate (WVTR) and Oxygen Transmission Rate (OTR)
Treatment |
OTR (g/m2.day) |
WVTR (g/m2.day) |
Chitosan film (n=6) |
54.867±0.033a |
2287.97±3.055a |
Chitosan-collagen composite film (n=6) |
0.012±0.000b |
2152.843±3.333b |
Chitosan-collagen nanocomposite film (n=6) |
0.006±1.998c |
1252.157±3.333 c |
Values are Mean±SD. There is no significant difference (p>0.05) between any two means within the same column that share the same superscript letter.
Table 5: Moisture content, swelling index, and water solubility
Treatment |
Moisture content (%) |
Degree of swelling (%) |
Water solubility (%) |
Chitosan film (n=6) |
36.800±0.611 a |
48.900±0.458 c |
14.713±0.043 a |
Chitosan-collagen composite film (n=6) |
31.033±0.448 b |
84.067±0.536 a |
12.800±0.058 b |
Chitosan-collagen nanocomposite film (n=6) |
23.463±1.27 b |
66.667±0.882 b |
10.130±0.091 c |
Values are Mean±SD. There is no significant difference (p>0.05) between any two means within the same column that share the same superscript letter.
.
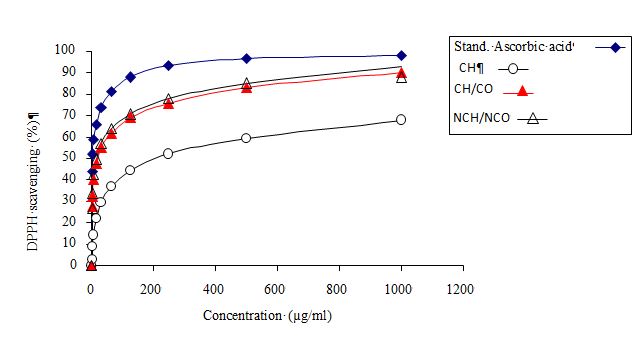
Figure 3: 2,2-Diphenyl-1-Picrylhydrazyl (DPPH) radical scavenging activity of edible films
Table 6: 2,2-Diphenyl-1-Picrylhydrazyl (DPPH) scavenging (%) of films
Concentration (µg/ml) |
Ascorbic acid(control) |
Chitosan film |
Chitosan/collagen
composite film |
Chitosan/collagen
nanocomposite film |
1000 |
98.29±0.13 aA |
67.83±0.14 aD |
89.95±0.14 aC |
92.60±0.07 aB |
500 |
96.53±0.06 bA |
59.32±0.10 bD |
83.13±0.11 bC |
84.94±0.13 bB |
250 |
93.39±0.20 cA |
52.03±0.21 cD |
75.79±0.03 cC |
78.22±0.11 cB |
125 |
87.93±0.07 dA |
44.60±0.20 dD |
69.17±0.22 dC |
70.95±0.10 dB |
62.5 |
81.20±0.09 eA |
36.84±0.12 eD |
61.88±0.01 eC |
64.14±0.12 eB |
31.25 |
73.67±0.13 fA |
29.56±0.19 fD |
54.82±0.02 fC |
56.83±0.10 fB |
15.625 |
66.25±0.11 gA |
21.80±0.14 gD |
47.93±0.05 gC |
49.90±0.06 gB |
7.8125 |
58.81±0.21 hA |
14.53±0.07 hD |
40.02±0.09 hC |
42.77±0.12 hB |
3.9 |
52.30±0.20 iA |
8.87±0.29 iD |
32.47±0.03 iC |
34.06±0.09 iB |
1.95 |
44.09±0.10 jA |
2.77±0.22 jD |
27.67±0.09 jB |
26.84±0.25 jC |
0 |
0.00±0.00 kA |
0.00±0.00 kA |
0.00±0.00 kA |
0.00±0.00 kA |
a, b, and c: There is no significant difference (p>0.05) between any two means within the same column that share the same superscript letter.
A, B, and C: There is no significant difference (p>0.05) between any two means within the same row that share the same superscript letter.
Table 7: Antimicrobial activity of films
Pathogenic microorganism |
Films |
Control |
Chitosan film |
Chitosan/collagen
composite film |
Chitosan/collagen
nanocomposite film |
Bacillus subtilis (ATCC 6633) |
27±0.58 bB |
*ND |
25±0.58 aC |
30±0.58 aA |
Staphylococcus aureus (ATCC 6538) |
24±0.30 cB |
*ND |
23±0.24 bC |
25±0.12 cA |
Salmonella typhi (ATCC 6539) |
20±0.56 dB |
*ND |
25±0.06 aA |
18±0.18 dC |
Pseudomonas aeruginosa (ATCC 90274) |
15±0.09 eC |
*ND |
16±0.12 dB |
18±0.12 dA |
Candida albicans (ATCC 10221) |
28±0.17 aA |
*ND |
22±0.09 cC |
26±0.12 bB |
Mucor circinelloid (AUMMC 11656) |
20±0.09 dA |
*ND |
ND* |
ND* |
a, b, and c: There is no significant difference (p>0.05) between any two means within the same column that share the same superscript letter.
A, B, and C: There is no significant difference (p>0.05) between any two means within the same row that share the same superscript letter.
*ND= Not Detectable
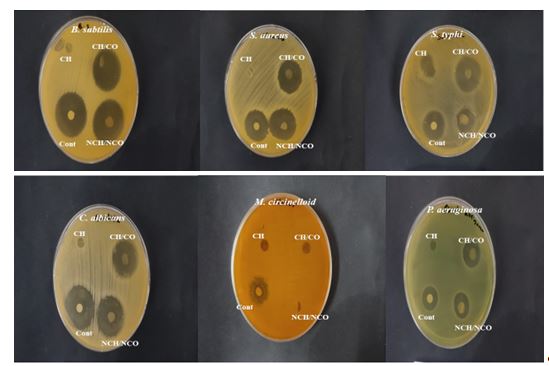
Figure 4: Antimicrobial activity (inhibition zone test) of chitosan film (CH), chitosan-collagen composite film (CH/CO), and chitosan-collagen nanocomposite film (NCH/NCO)
Collagen swell due to the weakening of binding ability between its interior molecular structure when pH is lowered to four or raised to 10 (Cheng et al., 2009). A recent investigation into collagen extraction from chicken feet revealed that a higher swelling capacity appears to be correlated with a higher collagen extraction yield capacity (Wahidin et al., 2021). Acetic acid has been reported as an excellent and highly effective solvent for collagen extraction (Apriliyani et al., 2020).
Appearance and transparency are very important factors for validating and evaluating food packaging materials. The recorded transmittance values are lower than 18.3%, indicating that the material is capable of absorbing UV rays up to 82% (Wahidin et al., 2021). These characteristics make it suitable for packaging foods sensitive to UV light, such as fruits and vegetables. The difference in transparency values between chitosan film, chitosan-collagen composite film, and chitosan-collagen nanocomposite film is caused by chitosan, which is colorless or has clear characteristics (Apriliyani et al., 2020). Therefore, the average transparency value in chitosan-collagen nanocomposite film decreases, followed by chitosan-collagen composite film. This may be due to differences in particle size between film components, the natural characteristics of the added active ingredients, or the thickness of the edible film. Nevertheless, the refractive index of the component materials has a greater impact on the transmittance value of the film than its thickness (Abd-Alhadi et al., 2023). In general, lighter film packaging is preferable to darker packaging, as it does not change the contents' natural color. However, when films are used to wrap foods susceptible to photo oxidation, the reduction in lightness may have a beneficial impact (Kishimoto, 2021; Kurek et al., 2021).
Film thickness is a sign of quality and the reliability of preparation process. According to Tang and Liu (2008), edible films with a thickness of about 0.071-0.083 ml can be classified as food coatings as their thickness is less than 0.25 ml, which commonly meets the requirements for food packaging. tensile strength measures a film's maximum resistance to applied tensile stress , whereas elongation% indicates how much it can stretch (Elsebaie et al., 2023). These interactions may improve bonding between chitosan-collagen composite film nanoparticles monomer in the gel film layer, and may result in tighter polymer chain-to-chain connections and increased resistance to mechanical stress.
Barrier properties of food packaging films, such as resistance to oxygen, carbon dioxide, water vapour, and organic vapor, are essentials in the prediction and assessment of packaged foods’ shelf-life (Shi et al., 2024). Water vapour permeability plays a significant role in food deterioration reactions. Conversely, adequate water content keeps food fresh and prevents dehydration. According to Rawdkuen et al. (2012), increasing film thickness can lead to greater water absorption from the environment. Moreover water vapour properties of chitosan films are influenced by their hydrophilic nature.
Oxygen plays an important role in the degradation reactions of various food, such as oxidation reactions, which can lead to vitamin loss, microbial growth, and changes in taste, color, aroma, etc. Furthermore oxygen permeability has a highly significant impact on the respiration of fresh vegetables and fruits (Shi et al., 2024). Our results may be clarified by the fact that collagen and nano-collagen compounds scattered on the composite film's surface altered the oxygen's diffusive path by occupying proper angles. Additionally Wang et al. (2016) suggested that penetrating molecules followed a more convoluted diffusive path, leading to a lower OTR. This may be explained by collagen molecules distributed on chitosan film, which occupied vertical oxygen diffusion channels, thereby extending the diffusion path and reducing oxygen permeability. Moisture absorption is a key component of biocompatible nanocomposite films used in food packaging. Films that absorb less moisture are better at preserving food, while higher water solubility can induce film biodegradability by shortening the film’s degradation period. However, high water solubility limits their application for foods with high water content (Siripatrawan and Harte, 2010). The high moisture content and water solubility of chitosan films can be attributed to their hydrophilic nature.
Edible films' swelling capacity provides valuable insights into their biodegradability and suitability for packing foods with higher moisture content, such as peeled fruits (Cerqueira et al., 2012). The significant decrease in the swelling index of chitosan film may be attributed to the inherent properties of chitosan, which is not easily soluble in water and it contains cationic groups (amines) and acetyl groups. The cationic groups contribute to the formation of strong and tight films, while the hydrophobic nature of acetyl groups further reduce water interaction (Apriliyani, et al., 2020). The increasing swelling in films with the addition of collagen (chitosan-collagen composite film), may be related to the hydrophilic characteristic of collagen or microstructure changes during drying. Additionally, lipid droplets alter the films' interior structure, causing water molecule migration. Conversely, the lower swelling index of chitosan-collagen nanocomposite film may be due to the nanoparticles, which act as highly effective agents in heal the colloidal particles migration through the medium (Sameni et al., 2015).
The antioxidant activity of edible films was evaluated using DPPH radical scavenging activity. chitosan-collagen nanocomposite film exhibited the highest antioxidant activity, followed by chitosan-collagen composite film, and then chitosan film. Our results are similar to those reported by López-Mata et al. (2015), which evaluated that chitosan has antioxidant properties due to the presence of nitrogen located at the C2 of the polymeric structure. Additionally, Nurilmala et al. (2020) observed that collagen peptides with lower molecular weight, ranging from 2.94 to 11.93 kDa, demonstrated stronger scavenging activity. High antioxidant activity (IC50) of collagen peptides were fractions of <3, 3-10, and 10-30 kDa. The smother nano-antioxidant properties of nanoparticles depend on their chemical composition, nature, stability, surface-to-volume ratio, size, surface coating, surface charge, and synthesized method. Moreover, the mechanism of nanoparticles as antioxidant may be due to free radicals quenching or the ability of the nanomaterial to convert alkyl peroxyl radicals into hydroperoxides by quenching them (Samrot et al., 2020; Valgimigli et al., 2018).
As described by Berechet et al. (2023) and Lima et al. (2015), collagen hydrolysates can be used as antioxidant and antimicrobial agents. The limited activity of chitosan film was noted by Pranoto et al. (2005), who found that chitosan film without any additives exhibited no inhibition zones against S. typhymurium and S. aureus. This result can be explained by the finding of Aranaz et al. (2021) and Hosseini et al. (2008), who reported that chitosan has the inherent characteristic of limited migrating. Additionally, chitosan doesn't diffuse through adjacent agar media, and only microorganism in direct contact with the active sites of chitosan are inhibited. Similarly, Aider (2010) found that variables such as the amount of water in the agar ,molecule size, shape, and polarity are related to migration.
The antimicrobial activity of chitosan-collagen nanocomposite film may result from the penetration of nanoparticles into the cell, as well as the production of hydroxyl (OH) and superoxide (O2) radicals. These radicals cause destruction of the bacterial cell membrane, interact with compounds in the cytoplasm, and ultimately lead to the death of viruses, bacteria, and fungi. Cell penetration is often the initial step in microbial inhibition before other mechanisms take effect. The main penetration mechanisms involve diffusion or adsorption of nanoparticles at the cell surface. Adsorption may occur through the binding of nanoparticles to negatively charged functional groups of proteins, resulting in protein destruction and cell death, or through interactions between various surface-exposed microbial groups and nanoparticles (Padmavathy and Vijayaraghavan, 2011; Sharmin et al., 2021).
Conclusion
In conclusion, this investigation clearly demonstrates that using chicken feet collagen hydrolyzed with 5% acetic acid to produce chitosan-collagen nanocomposite film is both successful and economically feasible. The prepared film exhibited favorable characteristics, including good mechanical, physical, antioxidant, and antimicrobial properties, as well as low oxygen permeability. These qualities make it suitable for use as packaging material, particularly for preserving animal-based or high-fat foods. For future work, the authors strongly recommend further studies on the beneficial effects of chitosan-collagen nanocomposites and hydrolyzed collagen in pharmacology and the food industry.
Author contributions
M.A.S. designed the study and reviewed the manuscript; M.A.S., N.S.M., and K.Y.F. conducted the experiments, performed the data analysis, and wrote the manuscripts. All authors read and approved the final manuscript.
Acknowledgements
The authors acknowledge the contribution of Dr. Yehia Heikal, Professor of Food Engineering at Ain Shams University, Cairo, Egypt, in reviewing the manuscript.
Conflicts of Interest
The author declares no conflict of interest regarding the publication of this research.
Funding
This manuscript received no specific grant from any funding agency in the public, commercial, or non-profit sectors
Ethical consideration
Not applicable.
References
Abd-Alhadi R., Abou-Ghorrah S., Al Oklah B. (2023). Physical properties, antioxidant and antimicrobial activity of chitosan edible films containing essential oils. Journal of Nutrition and Food Security.8: 212-220. [DOI: 10.18502/jnfs.v8i2.12595]
Aider M. (2010). Chitosan application for active bio-based films production and potential in the food industry: review. LWT - Food Science and Technology. 43: 837-842. [DOI: 10.1016/j.lwt.2010.01.021]
Amirrah I.N., Lokanathan Y., Zulkiflee I., Wee M.F.M.R., Motta A., Fauzi M.B. (2022). A comprehensive review on collagen type I development of biomaterials for tissue engineering: from biosynthesis to bioscaffold. Biomedicines. 10: 2307. [DOI: 10.3390/biomedicines10092307]
Ananth K.P., Paul P.K., Paliwal H., Nath V., Nakpheng T., Srichana T.(2024). Controlled degradation and kinetics response in calcium silicate doped with sodium alginate/functionalized multi-walled carbon nanotube composite 3D scaffolds for cartilage regeneration. Journal of Materials Research and Technology. 29: 4978-4990. [DOI: 10.1016/j.jmrt.2024.02.178]
Apriliyani M.W., Rahayu P.P., Andriani R.D., Manab A., Purwadi, Sawitri M.E., Utama D.T. (2020). Characteristics of casein–chitosan edible coating and its preservative effect in meat during accelerated storage. IOP Conference Series: Earth and Environmental Science. 478: 012060. [DOI: 10.1088/1755-1315/478/1/012060]
Aranaz I., Alcántara A.R., Civera M.C., Arias C., Elorza B., Caballero A.H., Acosta N.(2021).Chitosan: an overview of its properties and applications. Polymers. 13: 3256. [DOI: 10.3390/polym13193256]
Azizah F., Nursakti H., Ningrum A., Supriyadi. (2023).Development of edible composite film from fish gelatin-pectin incorporated with lemongrass essential oil and its application in chicken meat. Polymers. 15: 2075. [DOI: 10.3390/polym15092075]
Berechet M.D., Gaidau C., Nešić A., Constantinescu R.R., Simion D., Niculescu O., Stelescu M.D., Sandulache I., Râpă M. (2023). Antioxidant and antimicrobial properties of hydrolysed collagen nanofibers loaded with ginger essential oil. Materials. 16: 1438. [DOI: 10.3390/ma16041438]
Castaldo L., Izzo L., De Pascale S., Narváez A., Rodriguez-Carrasco Y., Ritieni A. (2021). Chemical composition, in vitro bioaccessibility and antioxidant activity of polyphenolic compounds from nutraceutical fennel waste extract. Molecules. 26: 1968. [DOI: 10.3390/ molecules26071968]
Cerqueira M.A., Souza B.W.S., Teixeira J.A., Vicente A.A. (2012). Effect of glycerol and corn oil on physicochemical properties of polysaccharide films - a comparative study. Food Hydrocolloids. 27: 175-184. [DOI: 10.1016/j.foodhyd. 2011.07.007]
Cheng F.-Y., Hsu F.-W., Chang H.-S., Lin L.-C., Sakata R. (2009). Effect of different acids on the extraction of pepsin-solubilised collagen containing melanin from silky fowl feet. Food Chemistry. 113: 563-567. [DOI: 10.1016/j.foodchem.2008.08.043]
Chuaychan S., Benjakul S., Kishimura H. (2015) .Characteristics of acid- and pepsin-soluble collagens from scale of seabass (Lates calcarifer). LWT - Food Science and Technology. 63: 71-76. [DOI: 10.1016/j.lwt.2015.03.002]
Dalle Zotte A., Ricci R., Cullere M., Serva L., Tenti S., Marchesini G. (2020). Research note: effect of chicken genotype and white striping–wooden breast condition on breast meat proximate composition and amino acid profile. Poultry Science. 99: 1797-1803. [DOI: 10.1016/j.psj.2019.10.066]
Delavari M.M., Ocampo I., Stiharu I. (2022). Optimizing biodegradable starch-based composite films formulation for wound-dressing applications. Micromachines. 13: 2146. [DOI: 10.3390/mi13122146]
Elsebaie E.M., Mousa M.M., Abulmeaty S.A., Shaat H.A.Y., Elmeslamy S.A.-E., Asker G.A., Faramawy A.A., Shaat H.A.Y., Abd Elrahman W.M., Eldamaty H.S.E., Abd Allah A.L., Badr M.R. (2023). Chitosan-based green pea (Pisum sativum L.) pod extract gel film: characterization and application in food packaging. Gels. 9: 77. [DOI: 10.3390/gels9020077]
Fatima S., Mir M.I., Khan M.R., Sayyed R.Z., Mehnaz S., Abbas S., Sadiq M.B., Masih R. (2022). The optimization of gelatin extraction from chicken feet and the development of gelatin based active packaging for the shelf-life extension of fresh grapes. Sustainability. 14: 7881. [DOI: 10.3390/su14137881]
González-Palma I., Escalona-Buendía H.B., Ponce-Alquicira E., Téllez-Téllez M., Gupta VK., Díaz-Godínez G., Soriano-Santos J. (2016). Evaluation of the antioxidant activity of aqueous and methanol extracts of Pleurotus ostreatus in different growth stages. Frontiers in Microbiology. 7: 1099. [DOI: 10.3389/fmicb.2016.01099]
Haghighi H., Licciardello F., Fava P., Siesler H.W., Pulvirenti A. (2020). Recent advances on chitosan-based films for sustainable food packaging applications. Food Packaging and Shelf Life. 26: 100551. [DOI: 10.1016/j.fpsl.2020.100551]
Hashim P., Mohd Ridzwan M.S., Bakar J. (2014). Isolation and characterization of collagen from chicken feet. World Academy of Science, Engineering and Technology International Journal of Bioengineering and Life Sciences. 8: .250-254. [DOI: 10.5281/zenodo.1091484]
Hosseini M.H., Razavi S.H., Mousavi S.M.A., Yasaghi S.A.S.Y., Hasansaraei A.G. (2008). Improving antibacterial activity of edible films based on chitosan by incorporating thyme and clove essential oils and EDTA. Journal of Applied Sciences. 8: 2895-2900. [DOI: 10.3923/jas.2008.2895.2900]
Hromiš N., Lazić V., Popović S., Markov S.,Vaštag Ž., Šuput D., Bulut S., Tomović V.
(2016). Investigation of a product-specific active packaging material based on chitosan biofilm with spice oleoresins. Journal of Food and Nutrition Research. 55: 78-88
Kishimoto N.(2021). Light protection performance of wrapping films to prevent the photo-oxidation of extra virgin olive oil during storage in glass bottles. AIMS Agriculture and Food. 6: 786-796. [DOI: 10.3934/agrfood.2021047]
Kurek M., Benbettaieb N., Ščetar M., Chaudy E., Repajić M., Klepac D.,Valić S., Debeaufort F., Galić K. (2021). Characterization of food packaging films with blackcurrant fruit waste as a source of antioxidant and color sensing intelligent material. Molecules. 26: 2569. [DOI: 10.3390/molecules26092569]
Lima C.A., Campos J.F., Lima Filho J.L., Converti A., Da Cunha M.G.C., Porto A.L. (2015). Antimicrobial and radical scavenging properties of bovine collagen hydrolysates produced by Penicillium aurantiogriseum URM 4622 collagenase. Journal of Food Science and Technology. 52: 4459-66. [DOI: 10.1007/s13197-014-1463-y]
Liu D.C., Lin Y.K., Chen M.T. (2001). Optimum condition of extracting collagen from chicken feet and its characteristics. Asian-Australasian Journal of Animal Sciences. 14: 1638-1644. [DOI: 10.5713/ajas.2001.1638]
López-Mata M.A., Ruiz-Cruz S., Silva-Beltrán N.P., Ornelas-Paz J.D.J., Ocaño-Higuera V.M., Rodríguez-Félix F., Cira-Chávez L.A., Del-Toro-Sánchez C.L., Shirai K. (2015). Physicochemical and antioxidant properties of chitosan films incorporated with cinnamon oil. International Journal of Polymer Science. 25. [DOI: 10.1155/2015/974506]
Mokrejs P., Gal R., Janaeova D., Plakova M., Zacharova M. (2017). Chicken paws by-products as an alternative source of proteins. Oriental Journal of Chemistry. 33: 2209-2216. [DOI: 10.13005/ojc/330508]
Momtaz M., Momtaz E., Mehrgardi M.A., Momtaz F., Narimani T., Poursina F. (2024). .Preparation and characterization of gelatin/chitosan nanocomposite reinforced by NiO nanoparticles as an active food packaging. Scientific Reports. 14: 519. [DOI: 10.1038/s41598-023-50260-8]
Ningrum A., Perdani A.W., Supriyadi, Munawaroh H.S.H., Aisyah S., Susanto E. (2021). Characterization of tuna skin gelatin edible films with various plasticizers-essential oils and their effect on beef appearance. Journal of Food Processing and Preservation. 45: e15701. [DOI: 10.1111/jfpp.15701]
Nurilmala M., Hizbullah H.H., Karnia E., Kusumaningtyas E., Ochiai Y. (2020). Characterization and antioxidant activity of collagen, gelatin, and the derived peptides from yellowfin tuna (Thunnus albacares) skin. Marine Drugs. 18: 98. [DOI: 10.3390/md18020098]
Okerman H.W. (1984). Quality control of post-mortem muscle tissue. Microbiology. 12th edition. The Ohio State University, Ohio, USA.
Ozturk-Kerimoglu B., Heres A., Mora L., Toldrá F. (2023). Antioxidant peptides generated from chicken feet protein hydrolysates. Journal of The Science of Food and Agriculture. 103: 7207-7217. [DOI: 10.1002/jsfa.12802]
Padmavathy N., Vijayaraghavan R. (2011). Interaction of ZnO nanoparticles with microbes—a physio and biochemical assay. Journal of Biomedical Nanotechnology. 7: 813-822. [DOI: 10.1166/jbn.2011.1343]
Pranoto Y., Rakshit S.K., Salokhe V.M. (2005). Enhancing antimicrobial activity of chitosan films by incorporating garlic oil, potassium sorbate and nisin. LWT - Food Science and Technology. 38: 859-865. [DOI: 10.1016/j.lwt.2004.09.014]
Rawdkuen S., Suthiluk P., Kamhangwong D., Benjakul S. (2012). Mechanical, physico-chemical, and antimicrobial properties of gelatin-based film incorporated with catechin-lysozyme. Chemistry Central Journal. 6: 131. [DOI: 10.1186/1752-153X-6-131]
Sameni A., Pourafshary P., Ghanbarzadeh M., Ayatollahi s.(2015). Effect of nanoparticles on clay swelling and migration. Egyptian Journal of Petroleum. 24: 429-437. [DOI: 10.1016/j.ejpe.2015.10.006]
Samrot V.A., Singh S.P.R., Deenadhayalan R., Rajesh V.V., Padmanaban S., Radhakrishnan R. (2020). Nanoparticles, a double-edged sword with oxidant as well as antioxidant properties—a review. Oxygen. 2: 591-604. [DOI: 10.3390/oxygen2040039]
Santana J.C.C., Gardim R.B., Almeida P.F., Borini G.B., Quispe A.P.B., Llanos S.A.V., Heredia J.A., Zamuner S., Gamarra F.M.C., Farias T.M.B., HO L.L., Berssaneti F.T. (2020). Valorization of chicken feet by-product of the poultry industry: high qualities of gelatin and biofilm from extraction of collagen. Polymers. 12: 529. [DOI: 10.3390/polym12030529]
Sharkawy A., Barreiro M.F., Rodrigues A.E. (2021). New pickering emulsions stabilized with chitosan/collagen peptides nanoparticles: Synthesis, characterization and tracking of the nanoparticles after skin application. Colloids and Surfaces A: Physicochemical and Engineering Aspects. 616: 126327. [DOI: 10.1016/j.colsurfa.2021.126327]
Sharmin S., Rahaman M., Sarkar C., Atolani O., Islam M.T., Adeyemi O.S. (2021). Nanoparticles as antimicrobial and antiviral agents: a literature-based perspective study. Heliyon. 7: e06456. [DOI: 10.1016/j.heliyon.2021.e06456]
Shi B., Hao Z., Du Y., Jia M., Xie S. (2024). Mechanical and barrier properties of chitosan-based composite film as food packaging: a review. BioResources. 19: 4001-4014. [DOI:10.15376/biores.19.2.Shi]
Siripatrawan U., Harte B.R. (2010). Physical properties and antioxidant activity of an active film from chitosan incorporated with green tea extract. Food Hydrocolloids. 24: 770-775. [DOI: 10.1016/j.foodhyd.2010.04.003]
Steel R.G.D., Torrie J.H., Dickey D.A. (1997). Principles and procedures of statistics: a biometric approach. 3rd edition. McGraw-Hill Book Company, Inc, New York, USA.
Tang C., Liu H. (2008). Cellulose nanofiber reinforced poly(vinyl alcohol) composite film with high visible light transmittance. Composites Part A: Applied Science and Manufacturing. 39: 1638-1643. [DOI: 10.1016/j.compositesa.2008.07.005]
Valgimigli L., Baschieri A., Amorati R. (2018). Antioxidant activity of nanomaterials. Journal of Materials Chemistry. B. 6: 2036-2051. [DOI: 10.1039/c8tb00107c]
Wahidin M., Srimarlita A., Sulaiman I., Indarti E. (2021). Transparency and thickness of jackfruit and durian seed starch edible film. IOP Conference Series: Earth and Environmental Science. 667: 012030. [DOI: 10.1088/1755-1315/667/1/012030]
Wang H., Hu D., Ma Q., Wang L. (2016). Physical and antioxidant properties of flexible soy protein isolate films by incorporating chestnut (Castanea mollissima) bur extracts. LWT - Food Science and Technology. 71: 33-39. [DOI: 10.1016/j.lwt.2016.03.025]
Wang H., Qian J., Ding F. (2018). Emerging chitosan-based films for food packaging applications. Journal of Agricultural and Food Chemistry. 66: 395-413. [DOI: 10.1021/acs.jafc.7b04528]
Youssef A.M., El-Sayed H.S., EL-Nagar I., El-Sayed S.M. (2021). Preparation and characterization of novel bionanocomposites based on garlic extract for preserving fresh Nile tilapia fish fillets. RSC Advances. 11: 22571-22584. [DOI: 10.1039/ d1ra03819b]
* Corresponding author (M.A. Sheir)
* E-mail: Marwa.sheir@yahoo.com
ORCID ID: https://orcid.org/0000-0001-7377-6107
Type of Study:
Original article |
Subject:
Special Received: 24/07/07 | Accepted: 25/02/02 | Published: 25/03/30